How to Copy Dna Over and Over Again
Abstruse
Analogous to a photocopier, we developed a Dna microarray copy technique and were able to copy patterned original DNA microarrays. With this process the advent of the copied Deoxyribonucleic acid microarray can as well be altered compared to the original by producing copies of dissimilar resolutions. As a homage to the very first photocopy fabricated by Chester Charlson and Otto Kornei, we performed a lookalike Dna microarray copy exactly 80 years later. Those copies were too used for label-complimentary existent-time kinetic binding assays of apo-dCas9 to double stranded Deoxyribonucleic acid and of thrombin to unmarried stranded DNA. Since each Deoxyribonucleic acid microarray copy was made with only v µl of spPCR mix, the whole procedure is price-efficient. Hence, our Dna microarray copier has a neat potential for condign a standard lab tool.
Introduction
Deoxyribonucleic acid microarrays are small structures on surfaces which are comprised of hundreds to thousands of individual DNA spots. They can exist used for a variety of applications, such every bit gene expression profiling, transcription factor binding assays and genotypingone,ii. Still, the generation of microarrays is yet cumbersome. Up to at present, the ii primary methods to produce them are spottingiii and in-situ lite directed synthesis (photolithography)4. Either way, the arrays are manufactured from scratch. For Dna microarray spotting, the private DNA sequences demand to be chemically synthesized or produced via cell culture in accelerate. However, when choosing chemical synthesis, the Dna length is limited to short oligomers because of increasing synthesis errors. Hence, for longer Deoxyribonucleic acid sequences, cell cultures and a high effort of DNA cloning are often unavoidable. This fact makes the creation of microarrays with many spots expensive. Using photolithography, the Dna microarrays are directly synthesized onto the chip. Consequently, only the four unlike Deoxyribonucleic acid nucleotides are needed. However, lithographic Dna microarrays are even more strongly restricted by the aforementioned sequence length limitations than chemical DNA synthesis. Hence, it is only useful for applications working with brusque Deoxyribonucleic acid sequences2.
Summing upwardly, edifice Deoxyribonucleic acid microarrays from scratch every time is expensive, fourth dimension consuming and often needs loftier-tech equipment to achieve the necessary reliability. However, if a DNA template is already available, the most effective fashion to replicate these Dna molecules is through the natural Dna replication reaction catalysed by Dna polymerases. The speed and precision of DNA polymerases are however unrivalled by chemical synthesis and will remain very much so for a long time5. Previous works have already demonstrated that polymerases tin can be utilized to replicate Dna microarrays6,7,8,9,10,eleven,12,13. However, none of them were able to perform a copy from a glass substrate to another glass substrate. Yet, since DNA microarrays are often spotted or synthesized onto drinking glass, this can be an obstruction. Furthermore, these replication techniques are based on the de-hybridization of complementary Dna molecules. This but allows the generation of a complementary Deoxyribonucleic acid microarray, representing a negative of the original DNA array.
In this piece of work, we depict an culling technique to copy original Dna microarrays, which is comparable to that of a standard office copier. The original DNA microarrays used were produced by classic spotting and are comprised of many fiddling spots. During the copy process, those spots become pixelated and the smallest feature which is transferred to the copy is defined as a dot. Hence, the original Dna microarrays are comprised of spots, whereas the copied Dna microarrays consist of dots. Depending on the applied copy resolution, one spot of an original microarray can consequence in multiple dots in the respective microarray copy.
Results
Microarray copying of printed Dna microarrays
We used solid phase polymerase chain reaction (spPCR) to replicate the DNA and a cavity chip to maintain the spatial order in our Dna microarray copying process. In a first spPCR the DNA from the original DNA assortment is copied into cavities (Fig. 1a–c) and in a 2d spPCR the Deoxyribonucleic acid is copied back from the cavities onto a new surface (Fig. 1d–f). The resulting DNA microarray is now a copy of the original microarray consisting of the same DNA and comprising the same spatial data. The spPCR reaction used in our experiments is like to Hoffman et al. 2012 (Fig. 1g). Moreover, the surface primer used to amplify the Deoxyribonucleic acid molecules on the surface were immobilised with help of the well described PDITC chemistry (Fig. 1h)14,xv.
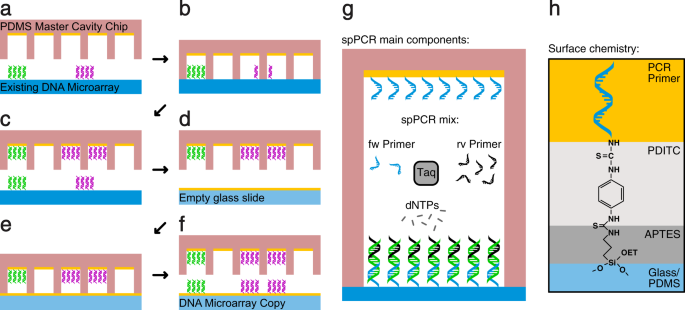
The principle of DNA microarray copying. (a) A PDMS chief crenel chip coated with primer is filled with spPCR mix and placed on top of an original DNA microarray consisting of two different DNA species (magenta and dark-green). (b) Subsequently closing, a kickoff spPCR is performed to amplify the DNA and (c) to attach it to the within of the cavities. (d) The cavity chip is washed, blocked, and refilled with fresh spPCR mix. (e) The crenel chip is placed on summit of an empty glass slide coated with primer and a second spPCR is performed. (f) Later the spPCR, the cavity chip is opened, revealing a copy of the original Deoxyribonucleic acid microarray. The position, size and number of the cavities limits the spatial resolution of the copy. In this example, the dark-green DNA spot is enclosed by one cavity resulting in one dot in the copy. The magenta Deoxyribonucleic acid spot is enclosed by two cavities, hence creating ii magenta Deoxyribonucleic acid dots. (g) Illustration of the spPCR components. One PCR primer species is attached to the surface. After an initial distension in liquid phase, the PCR reaction is forced to the surface. (h) Illustration of the chemic limerick of the surface. The individual layers are indicated using unlike colors.
To demonstrate the working principle of our DNA copier, we produced original Dna microarrays past printing a simple pattern consisting of two different single stranded Dna molecules (magenta: 89 bp; green: 103 bp). We accept called our spotting scheme to resemble the style of the first photocopy by Chester Charlson and Otto Kornei and performed the copy exactly lxxx years later. Similar to Charlson'south first copy (Fig. 2a), we spotted the date of our re-create experiment and the proper name of our constitute (Fig. 2b). We used PDMS master crenel chips that had approximately the same crenel dimensions as the spots of the original DNA arrays. Even so the overall chip area dimensions are larger (Supplementary Table S1). Hence, the chip was manually placed onto the surface area of the microarray without farther precautions most alignment. This scanning procedure results in a random alignment of private cavities to individual, original spots. Even without a perfect alignment, the image will exist recreated depending on the resolution (in this example the crenel number and diameters). After the re-create experiment, all DNA microarrays – the originals too as the copies – were hybridised using fluorescently labelled DNA probes, resulting in a 2-colour image for each assortment. In both illustrated copies (Fig. 2c,d) the engagement likewise equally the plant name are conspicuously readable. In addition, the arrangement of the green and magenta Deoxyribonucleic acid dots matches the spots of the original array (Fig. 2b). The measurement of the signal intensities of the copied dots revealed lower values by a factor of 3.98 ± 0.41 (n = iii) in the green and by a factor of 8.64 ± 2.55 (northward = 3) in the magenta channel (Supplementary Fig. S3). If original DNA spots and the cavities of the master cavity chip are in perfect alignment, a nearly identical spatial image is created (Fig. 2c). If the cavities of the chip are misaligned, a blurred but yet readable copy is generated (Fig. second). For a more than circuitous Deoxyribonucleic acid image, nosotros used the aforementioned DNA sequences to print smileys (Fig. 2e) and subsequently generated a re-create (Fig. 2f).
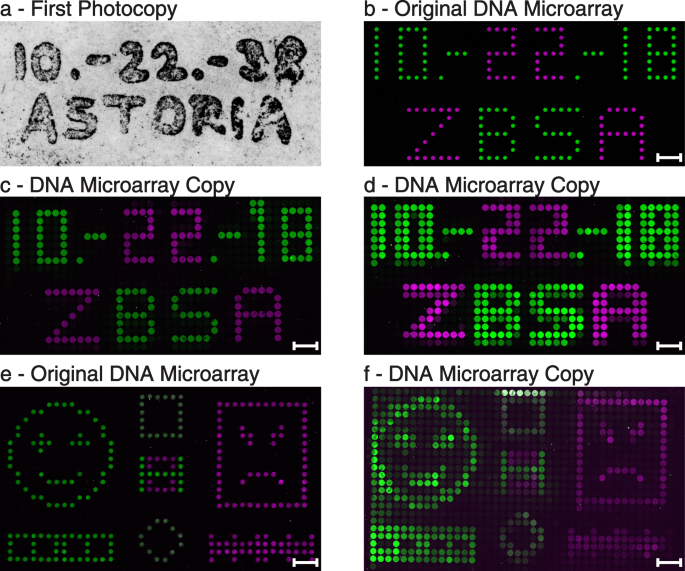
Examples of DNA microarray copies. (a) The very first photocopy made by Chester Charlson (Courtesy of Xerox Corporation). (b) Our lookalike spotted original DNA microarray containing date and plant name. (c) A DNA microarray copy of the original Dna microarray with a perfect alignment of Dna spots and cavities. (d) Another Deoxyribonucleic acid microarray copy, but with a misalignment of Dna spots and cavities, generating a blurred copy. (e) A spotted original DNA copy comprised of a happy and an angry smiley with additional structures. (f) The corresponding copy of the original smiley array. (c,d,f) Image contrasts were enhanced by a gene of 4 in the green channel and past a cistron of 7 in the magenta channel for better visualization. The scale confined show a length of 1 mm. All illustrated copies were produced using cavity bit two (Supplementary Table S1), with cavity diameters of 300 µm and a cavity distance of 50 µm. Spotted original spots have a diameter of 180 µm and a spot distance of 170 µm.
Resolution and moiré patterns
Moiré patterns are interference patterns which can be observed when a grid is placed on height of another gridxvi. Especially if the grids differ in spatial resolution or filigree pattern (e.k. quadratic vs. hexagonal), new shapes can exist created. The appearance of Moiré patterns can be used to generate DNA microarray copies with contradistinct dot patters compared to the one of the original DNA microarrays. For this purpose, we performed DNA microarray copies using cavity chips of college (i.3 times smaller cavity bore) as well as lower spatial resolution (ii.8 times larger crenel diameter) than the spots of the original Dna microarray (Supplementary Table S1). Again, nosotros used the aforementioned microarray blueprint as before (Fig. 2b). In case of the high-resolution copy (Fig. 3b), the cavities of the PDMS master cavity bit are bundled in a hexagonal, honeycomb-like pattern. Depending on the alignment of the cavity fleck on the original DNA microarray, each DNA spot generates a Moiré pattern of either vertical or flat rhombuses (Fig. 3b, enlarged sections). Hither, nigh four dots of the re-create are generated by one spot of the original DNA microarray. Choosing a lower resolution master cavity chip resulted in a smeared and blurred copy (Fig. 3c). Hither, near vii spots of the original array are depicted by five dots of the copy. The chosen spatial resolution of the crenel ensured that the original spots could non get in contact with more two cavities. Therefore, depending on how the master cavity scrap was aligned on the original DNA microarray, either one or ii larger DNA spots tin be observed in the corresponding re-create (Fig. 3c, enlarged sections).
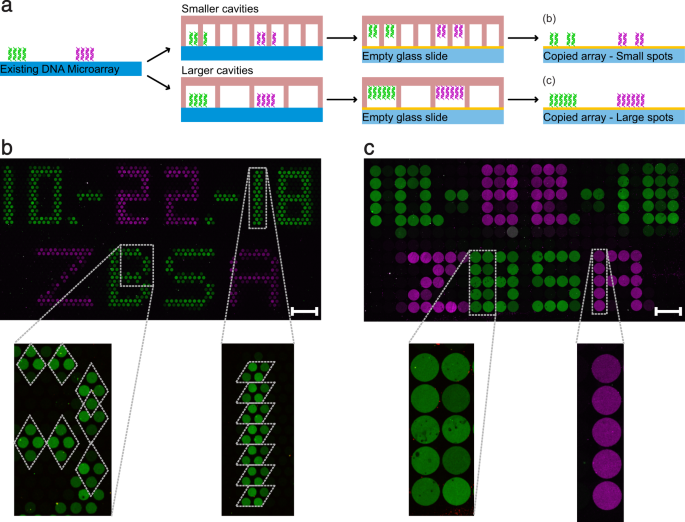
DNA microarray copies can as well be performed with altered resolutions. (a) Schematic representation of the performed microarray copy approach. The principles are similar to the method shown in Fig. 1a–f. However, this fourth dimension cavity chips were used with smaller (b) or larger (c) cavity diameters compared to the original spot sizes. (b) Hybridised fluorescent prototype of the pocket-size cavity (150 µm) re-create with enlarged sections. (c) Hybridised fluorescent image of the large cavity (500 µm) copy with enlarged sections. Dotted lines in the enlarged pictures illustrate the Moiré design. The scale bars prove a length of 1 mm. Cavity chip type 1 was used for the loftier resolution copy (b) and cavity chip blazon 3 was used for the lower resolution copy (c).
Dot merging
Depending on the original DNA microarray spot sizes, distances and the spatial resolution of the principal crenel chip, information technology is besides possible to merge spots on the Dna copy into i single Deoxyribonucleic acid dot. This transformation tin accept identify when ane cavity of the chief cavity flake is placed on top of ii spots of the original assortment. Hence, the spatial resolution of the master cavity chip has to exist identical to or lower than the resolution of the original assortment. This kind of abnormality tin exist exploited and even enforced in order to create Deoxyribonucleic acid mixture spots, which were not present on the original array. We generated an original DNA microarray via a digital PCR process with a hexagonal blueprint of round spots (diameter of 150 µm; distance of l µm), similar to a method published before15. Subsequently, we made a copy using a quadratic blueprint of round dots (bore of 300 µm; altitude of 50 µm) featuring an about 2 times lower resolution (Fig. 4a,b). The copied dots in the heart (Fig. 4a,b, right sides) contain a mixture of the magenta and the light-green coloured DNA species.
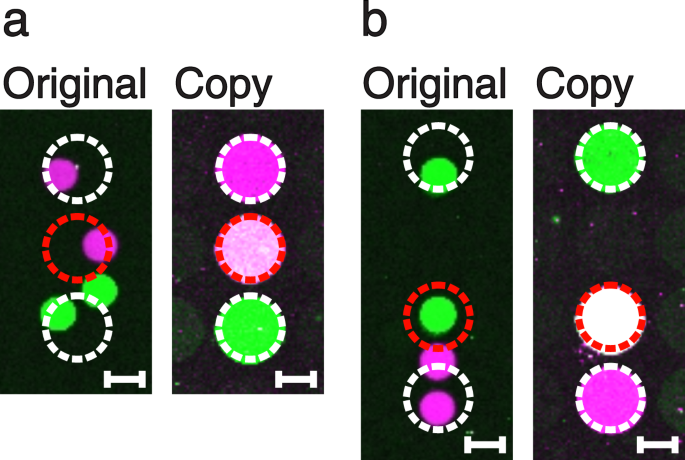
Ii examples of spot merging (a,b). The original Dna microarrays (left) were produced past digital solid stage PCR using our PDMS master cavity chips. Thereafter, copies of lower resolution were performed (right). In each case, the middle dots (red dashed circle) of the copies prove a pinkish to white colour, indicating that they are mixtures of both, the magenta and green DNA species. Calibration confined show a length of 100 µm.
Characterization-costless bounden experiments using copied Dna microarrays
Our spotted original microarrays are made of unmarried stranded Dna (ssDNA) molecules. By default, the copied Dna microarrays contain double stranded Deoxyribonucleic acid (dsDNA) every bit this is the natural end product of the spPCR. Still, using our surface chemistry, but ane of the 2 DNA strands is covalently bonded to the surface. Hence, the copies can exist de-hybridised in lodge to turn the dsDNA into ssDNA. Consequently, our copy approach allows united states of america to choose between dsDNA and ssDNA microarrays. This is a characteristic which is non yet hands accessible with any other microarray manufacturing process. However, having both types of Dna molecules available can be particularly useful for screening purposes. As an example, we used our copied microarrays and performed an apo-dCas9 (Cas9 not able to cut DNA without crRNA) assay with the dsDNA microarray (Fig. 5a) and a thrombin analysis with the ssDNA microarray (Fig. 5b). The sequences used for our re-create experiments (magenta and green spots) are derived from known and well-characterized thrombin aptamers17. Hence, in their unmarried stranded class they are able to bind to thrombin with a high specificity. Nosotros performed label-free single colour reflectometry (SCORE) cease point measurements to evaluate the binding of the proteins18. Additionally, we quantified the boilerplate bounden intensities of all magenta and dark-green spots on the microarray (in accord with Fig. 2b). Furthermore, four large areas exterior of the array region were analysed for obtaining a background betoken. The copied Deoxyribonucleic acid microarray showed a stronger apo-dCas9 binding than the corresponding original Dna microarray, including its groundwork (Fig. 5a). Moreover, all numbers and letters are clearly readable. No major differences in binding intensities for the magenta and dark-green spots could be observed within the original or copied assortment signals.
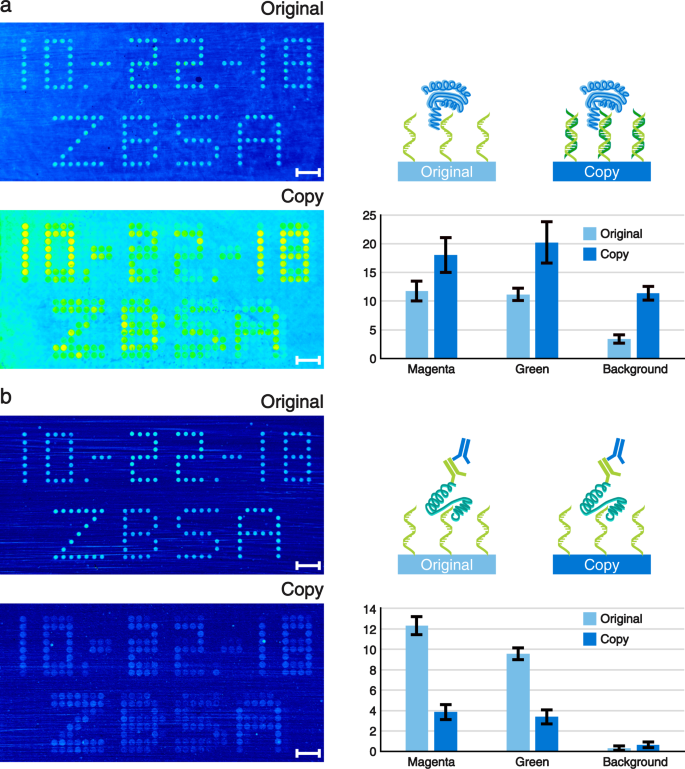
Label-gratis SCORE measurement pictures of (a) apo-dCas9 and (b) thrombin binding to the original and copied Deoxyribonucleic acid microarrays are shown on the left. The more yellow to red the spots and dots appear, the stronger is the binding signal. Corresponding schematic representations and bar plots illustrating the average binding signals of the spots are shown to the correct of each analysis. The units of the bar plots are given in milliSCORE. The apo-dCas9 assay was performed using the protein only, whereas for the thrombin measurement a iii step assay with thrombin followed by a principal and a secondary antibody was used. The bounden signals of the spots were averaged co-ordinate to their fluorescent colours (see Fig. 2). Background signals were assessed by measuring iv large background areas around the array region. The values of the bar plots represent the means and the error bars bear witness their standard deviations. N-values for (a): original-magenta = 244; original-green = 98; copy-magenta = 122; copy-green = 159. Northward-values for (b): original-magenta = 71; original-green = 98; copy-magenta = 59; copy-greenish = 183. Northward-values of all backgrounds equal 4. Calibration confined testify the length of one mm.
After the apo-dCas9 analysis, both Dna microarrays were de-hybridised to strip off all apo-dCas9 proteins and to generate a ssDNA array out of the dsDNA microarray re-create. Our thrombin SCORE experiment was performed in three steps. Commencement, thrombin was flushed over the DNA microarray, followed past a primary antibody, followed past a secondary antibody (Fig. 5b, schematic representation). The experiment revealed that in that location is indeed a good binding of thrombin and the corresponding antibodies to all spots of the original Deoxyribonucleic acid microarray (Fig. 5b). The formerly magenta coloured spots were quantified to show a slightly stronger bounden signal compared to the green spots. Our copied Deoxyribonucleic acid microarray also showed a binding signal to thrombin and the antibodies. However, the signal is weaker compared to the original microarray only however distinguishable from the background.
Since SCORE is a real-fourth dimension imaging technique to perform characterization-free binding experiments, we also provide videos of the measured bounden events (Supplementary Movies S4 and S5).
Give-and-take
The outset photocopy was made on the 22nd of Oct 1938, offer new possibilities to replicate texts and images. Exactly 80 years afterward, we performed a Dna copy, enabling us to copy a Dna epitome from an original DNA microarray. All microarrays were conspicuously readable and showed an exact effigy of its original paradigm (Supplementary Fig. S2). Although the copies showed a lower fluorescent signal than the original arrays, the DNA sequences remained identical. Since the copy of one Dna microarray only needs v µl of spPCR mix, the process is cost-efficient. In addition, all Dna microarray copies initially incorporate dsDNA, which can be converted into ssDNA microarrays after a de-hybridisation step. Since we make utilize of spPCR, a perfect unidirectional orientation of the DNA molecules with a 5′ (surface) to three′ direction can be ensured. Other photolithographic methods are also available with which such an orientation can be achieved, although a five′-three′ orientation is not standard19,20. The DNA density on the spot is mainly limited by the number of primers attached to the surface, the size of the polymerase and the number of spPCR cycles used. This allows many optimization and amending possibilities, for case an on-demand fine-tuning of the copied DNA spot densities, which is comparable to a brighter or darker photocopy.
Similar to a photocopy, we occasionally observed positive dots on the copied DNA microarrays where no template spot is encountered on the original. These errors may arise from cross contaminations which can occur prior to or during the spPCR. The first case tin be caused by loose Dna strands that are distributed on the original DNA microarray and may originate from bereft blocking or washing procedures. The 2d instance can occur through a leakage between neighbouring cavities. Furthermore, it can happen that no Deoxyribonucleic acid dot is observed where there should be one according to the original (no instance shown). Those effects mainly take place considering of tiny air bubbles trapped within the cavities of the PDMS master crenel chip.
We also demonstrated that our Deoxyribonucleic acid microarray copies underlie the same effects similar a pixelated photocopy, including spot merging and moiré patterns. Even so, these effects may not only happen accidentally, but tin can besides exist enforced through a careful selection of the cavity sizes and layouts. They can thus be used for Deoxyribonucleic acid synthesis, modifications or alterations of existing Deoxyribonucleic acid microarrays on purpose. Nosotros were able to prove that ii different spots of the original microarray tin can be combined on the microarray re-create. Hence, our method does not only allow the replication of existing DNA microarrays but also their alteration. We conclude, that new microarrays could be created past choosing the correct PDMS chief cavity bit dimensions. Depending on the correct choice of primer combinations, it should theoretically be possible to combine and mix 2 existing source microarrays. In principle, 2 Deoxyribonucleic acid microarrays would be copied on top of each other. This technique would allow to generate barcoded Deoxyribonucleic acid microarrays, which tin can then exist used for all kinds of transcriptomic studies. In theory, many known biochemical reactions for DNA tin can exist applied within the cavities of the master cavity flake to alter or modify the Deoxyribonucleic acid. Having those possibilities of DNA modifications (PCR, cloning, gene editing and many others) in mind, our copies may be the "newspaper" which many new high-throughput applications may be "written" on.
Our SCORE experiments demonstrated that the molecules of the copied DNA microarrays are accessible for label-free binding measurements. Although the copied Dna microarrays are comprised of less Dna molecules per dot than the original array, the apo-dCas9 binding signal was much stronger for the copied array. Since the copied array contains double stranded Deoxyribonucleic acid molecules after the copy process, we conclude that the apo-dCas9 protein has a higher specificity to dsDNA molecules than to ssDNA molecules. Interestingly, the groundwork of the copied Deoxyribonucleic acid microarrays is in the range of the magenta/light-green spots of the original array. This is because the whole microarray slide of the copy is homogenously covered with single stranded forward primer needed for the spPCR. That is not the example for the spotted microarray, which only consist of the Deoxyribonucleic acid spots. Therefore, the background of the copied Dna microarray basically displays the bespeak of apo-dCas9 binding to single stranded Dna molecules. The performed Thrombin analysis on the ssDNA microarray re-create showed a specific binding to the single stranded Deoxyribonucleic acid molecule aptamers. However, the Thrombin point of the copied array is lower compared to the original assortment for both DNA species, which is in consistence to the hybridization results. The differences between original and copy are still subject of research. Nonetheless, these label-free screening results show that our DNA microarray copy technique tin be used for multiple screening applications.
Our DNA microarray copy method combined with the label free SCORE measurement holds a great potential for old and new applications. We think that the presented DNA copies just represent the first step of microarray copying. Since the DNA molecules of the original DNA microarray are immobilised within the cavities of the master crenel bit, RNA21,22 or poly peptide23,24,25,26 copies could also be realised. This can be done past changing the re-create surface and biochemical mix. Such copies will open a new world of screening and synthetic biochemistry.
Material and Methods
Structuring of Si-wafers as primary mould
For realising the desired structures in PDMS, make clean standard 4″ Si-wafers were used. A wafer was heated to 130 °C for threescore south. The hot wafer was laminated as quickly every bit possible (ORDYL SY330 Elga Europe, Italy) according to manufacturer protocol using a Polatek laminator. Before exposure, the photolithography mask (Zitzmann GmbH, Germany with a resolution of 32,000 dpi) had to be placed in the maskaligner (MA6/BA6 Karl Suss). Masks were drawn using Solidworks (SW2013) and Coreldraw (CorelDraw X6 V16). The wafer was illuminated for 12.v s in soft contact way with an alignment-gap of 100 µm. Thereafter, the wafer was baked at 85 °C for 60 s. Next, the wafer was developed in ordyl-developer (Elga Europe, Italy) for about 20 southward, followed by another fifteen s launder in ordyl-developer. The developer times depend on the mask structures and need to be adjusted. After developing, the wafer was broiled at 100 °C for sixty min. Finally, the wafer was placed in a desiccator together with a microscope slide carrying a drop of 25 μl of Trichloro(1H,1H,2H,2H-perfluorooctyl)silane (Sigma-Aldrich, Germany) for 8 hours, followed past a post bake step of 60 min at 90 °C.
Moulding tools product
To prevent wafer damage, we developed in business firm moulding tools made out of aluminium. The wafers were glued to the hat using Soudal Fix ALL CRYSTAL glue (Soudal North. Five., Germany). After alignment, an overnight drying footstep was applied. The bottom part of the tool has placeholders for the aluminium carriers. Nosotros used backbone aluminium carriers with a thickness of 1 mm, a length of 75 mm and a width of 25 mm (same dimensions as a microscope slide). We likewise designed them to have holes for the later on fluidic connections.
PDMS crenel chip production
2 aluminium metal sheets (standard microscope glass slide measurements), containing two one mm holes, were plasma activated using a plasma generator (ZEPTO, Diener electronics) for 2 min at 100 W and at a gas flow of twenty l/h. PDMS (Elastosil RT 607; Wacker Chemie, Deutschland) was mixed in a 9:1 ratio (component A: component B) with a speedmixer (speedmixer Series DAC 150 for sixty s at 1500 rpm). Subsequently, the metal sheets were put into our moulding forms and coated with 9 g of even so liquid PDMS. The moulding form was put nether vacuum in a desiccator for vi min. Thereafter, the moulding form was closed with the lid which contains the structured wafer. Next, the airtight moulding form was incubated at threescore °C for ane h. Thereafter, the PDMS cavity chips were demoulded and microfluidic holes were punched into the PDMS using a 0.5 mm biopsy punch.
Production of primer coupled glass slides
Glass slides (standard microscope slides too as SCORE slides) were rinsed with acetone, isopropanol and diHiiO. Subsequently, they were dried in an Nii gas stream. Prior to silanisation, slides were plasma activated using a plasma generator (ZEPTO, Diener electronics) for 1 min at 100 W and at a gas menstruum of 20 50/h. Thereafter, slides were put into a (3-aminopropyl)triethoxysilane (APTES, Sigma-Aldrich, Germany) solution (89% acetone [v/five], 10% dH2O [5/v] and 1% APTES [5/v]) and incubated at RT for 30 min. Subsequently, slides were done 3 times for 5 min in acetone, dried in a N2 stream and incubated for 45 min at 110 °C. After incubation, slides were showtime cooled downwardly to RT using an Ntwo gas stream and so put into a p-phenylene diisothiocyanate (PDITC, Sigma-Aldrich, Germany) solution (10 mM PDITC in 90% DMF [5/5] and 10% pyridine [v/v]), followed past an incubation for two h at RT. Subsequently, the slides were flushed with ethanol and done two times in ethanol for 5 min, followed past acetone for 5 min. Slides were dried in a N2 stream and put under vacuum in a desiccator for 15 min. Thereafter, slides were primer coupled by incubation in a primer solution (150 mM NaH2PO4, 150 mM Na2HPO4, 200 nM aminated DNA primer (Biomers, Federal republic of germany)) at RT overnight. After incubation, slides were put into a blocking solution (x mg/ml BSA in h2o) for 5 min. And then, 5% [five/v] ethanolamin was added and slides were incubated for another 25 min. Subsequently, slides were rinsed with water several times and put into 70 °C hot water for 10 min. The hot water was exchanged and slides were incubated for 5 min at 70 °C, rinsed with water at RT and dried in an N2 gas stream.
Production of primer coupled PDMS master cavity fries
PDMS cavity chips were rinsed with seventy% denatured ethanol, dried in an Northward2 gas stream and put nether vacuum in a desiccator for 30 min. Thereafter, chips were plasma activated using a plasma generator (ZEPTO, Diener electronics) for one min at xxx W and at a gas flow of 20 50/h. Subsequently, chips were put into a (3-Aminopropyl)triethoxysilane (APTES) solution (xc% ethanol [v/v], 5% dH2O [v/v], v% APTES [v/v]) overnight. Afterwards incubation, chips were washed three times in ethanol for five min, dried in an N2 gas stream and incubated for 45 min at 70 °C. Next, chips were cooled downward to RT using an N2 gas stream and put into a p-phenylene diisothiocyanate (PDITC) solution (x mM PDITC in ninety% DMF [v/v] and 10% pyridine [v/v]) followed past an incubation at RT for two h. Thereafter, fries were done three times in ethanol for 5 min, dried in an N2 stream and put nether vacuum in a desiccator for 1 h. Later on incubation, chips were put out of vacuum one after another and filled with a primer solution (150 mM NaH2PO4, 150 mM Na2HPO4, two µM aminated Deoxyribonucleic acid primer (Biomers, Federal republic of germany)) by adding 10 µl of solution directly onto the crenel region. Cavity chips were closed using a clean, untreated microscope drinking glass slide and incubated at RT overnight. Afterward incubation chips were put into a blocking solution (10 mg/ml BSA in DI h2o) and put under vacuum until all cavities were properly filled with blocking solution. Subsequently, chips were taken out of the vacuum and incubated for 5 min. Then, 5% [v/5] ethanolamin was added and chips were incubated for another 25 min. Next, chips were rinsed with water several times and put into 70 °C hot water for x min. The hot water was exchanged and chips were incubated v min at lxx °C, rinsed with water at RT and stale in an N2 gas stream.
Deoxyribonucleic acid microarray re-create
PCR 1 - Scanning of the original DNA microarray
Primer coupled PDMS master cavity chips were filled with four.8 µl of spPCR mix (five U Taq-polymerase [QIAGEN, Germany], 1x Taq reaction buffer, 1.v mM MgCl2, 0.half dozen mM dNTPs [QIAGEN, Germany], 3 mg/ml BSA, 0.05% Tween 80 [v/5], 0.125 µM frontward Dna primer, ii µM reverse DNA primer) and sealed with a drinking glass slide containing the original Dna microarray. Next, the outer chip regions of the closed fries were filled with 50% glycerin. The microfluidic holes, as well as the backside region of the crenel region, were covered with 1 mm thick PDMS pieces. An untreated glass slide was placed onto the PDMS pieces and the whole copy flake sandwich was clamped between a minor, U-shaped aluminium holder, containing four plastic spring screws. Thereafter, a standard PCR was performed using three temperature regulated water baths (Julabo, Germany) for fifteen cycles. Then, the fleck stacks were opened and the chips too as the original DNA microarrays were washed in 5x SSC buffer, containing 0.one% SDS for 5 min, followed by a washing step in 0.1x SSC buffer for 5 min. Adjacent, the drinking glass slides were flushed with water and dried in an N2 gas stream. The PDMS master cavity chips were dehybridised at 95 °C for 5 min in a dehybridisation solution (50% urea [five/five], 0.v% Tween twenty, 340 mM NaCl in water). Afterward, chips were done with h2o and stale in an Nii gas stream.
PCR 2 - Blocking of the PDMS master cavity flake cavities
The whole process of PCR 1 was repeated in order to block all remaining primer on the PDMS chief cavity fleck. However, for this footstep a blocking spPCR mix (containing 2 µM DNA blocking oligo instead of primer) and normal, untreated glass slides instead of an existing microarray were used. The blocking oligos consisted of the sequence "CCCCCCATGCGGGGGGTAGGTCCTA", followed past the reverse complementary sequence of the primer on the crenel flake surface. After the PCR and disassembling of the chip stacks, the untreated glass slides used to close the cavities were thrown abroad instead of washed and stale.
PCR 3 - Dna microarray copy
The blocked and dehybridised PDMS master cavity fries were put under vacuum in a desiccator for thirty min. Then, the cavity chips were taken out of the vacuum one after some other, cavities were filled with 4.viii µl of spPCR mix and sealed with primer coupled drinking glass slides. These slides contained the same forward PCR primer sequences attached to their surfaces as those used in the spPCR mix. Again, the airtight chips were filled with l% glycerin and the microfluidic holes, every bit well as the backside region of the cavity region, were covered with 1 mm thick PDMS pieces. An untreated glass slide was placed onto the PDMS pieces and the whole copy chip sandwich was clamped between a pocket-sized, U-shaped aluminium holder, containing 4 plastic leap screws. Thereafter, a standard PCR was performed using three temperature regulated water baths for 15 cycles. So, the scrap stacks were opened and the chips too every bit the original DNA microarrays were washed in 5x SSC buffer, containing 0.1% SDS [v/five], for 5 min, followed past a washing step in 0.1x SSC buffer for five min. Side by side, the fries and glass slides, which then were a copy of the original DNA microarray, were rinsed with water and dried under an Nii gas stream.
Glass slide hybridisation and fluorescent measurement
Drinking glass slides were washed in 5x SSC buffer, containing 0.1% SDS [5/5], for 5 min, followed by a washing step in 0.1x SSC buffer for 5 min. Subsequently, slides were dehybridised in dehybridisation solution (fifty% urea [5/v], 0.5% Tween 20, 340 mM NaCl in water) for 5 min at 95 °C, followed by a washing step in water for 5 min at RT. Next, slides were hybridized (5x SSC buffer, 0.1% SDS, 10 nM Cy5 labelled DNA probe (Biomers, Deutschland), 10 nM Cy3 labelled DNA probe (Biomers, Germany)) for 5 min at 95 °C. Subsequently, slides were put to xl °C together with the hybridisation solution for x min. Thereafter, slides were washed in 2x SSC, containing 0.ane% SDS [5/five], for 3 min at 40 °C, followed by a washing pace in 1x SSC for 5 min at 40 °C. Adjacent, slides were rinsed in water and dried in an Nii gas stream. Subsequently, fluorescent signals of the hybridized DNA probes were analysed in a GenePix 4000B microarray scanner using GenePix software version 7 Pro. All further analyses regarding spot intensities were made using ImageJ one.51 s.
Dna binding analysis
All SCORE experiments were performed with our in-house build SCORE car, which was previously published18. The exposure time of the camera was set to 19 ms with an image averaging of 64. Nosotros used a flow cells made of PDMS with a microfluidic gap of 30 μm and a full volume of 25 μl. Experiments were analysed using Anabel27.
Cas9 assay
The slides were blocked with 1 ml of 10 mg/ml BSA in Cas9 reaction buffer (20 mM HEPES, 0.1 1000 NaCL, 5 mM MgCltwo, 0.1 mM EDTA, pH vi.5) for 10 min. Later washing with DI water, the slides were dried in an North2 stream and inserted into the SCORE setup. The post-obit microfluidic assay sequence was performed: (1) Cas9 reaction buffer (300 s; threescore μl/min); (2) 10 mg/ml BSA in Cas9 reaction buffer (300 south; 60 μl/min); (3) Cas9 reaction buffer (300 s; 60 μl/min); (4) 48 ng/µl apo-dCas9 in Cas9 reaction buffer (400 southward; lx μl/min); (5) Cas9 reaction buffer (900 southward; threescore μl/min)
Thrombin assay
The slides were de-hybridised (50% urea [5/5], 0.5% Tween 20, 340 mM NaCl) for five min at 95 °C. Thereafter, slides where blocked with 1 ml of 10 mg/ml BSA in BBKC buffer (xx mM TRIS, 100 mM NaCl, 2 mM MgCl2, five mM KCl, 1 mM CaCl2, 0.02% Tween20 [v/v], pH 7.6) for ten min. Later on washing with DI h2o, the slides were stale in an Ntwo stream and inserted into the SCORE setup. The following microfluidic assay sequence was performed: (i) BBKC buffer (300 s; lx μl/min); (2) ten mg/ml BSA in BBKC buffer (300 due south; 600 μl/min); (iii) BBKC buffer (300 s; 60 μl/min); (4) x µg/ml Thrombin (Sigma-Aldrich, Frg) (300 s; 60 μl/min); (v) BBKC buffer (600 south; 60 μl/min); (6) x µg/ml anti-thrombin antibody (ab20877, Abcam, Germany) (300 due south; 60 μl/min); (vii) BBKC buffer (200 s; sixty μl/min); (8) x µg/ml secondary antibiotic (ab150110, Abcam, Federal republic of germany) (300 south; threescore μl/min); (9) BBKC buffer (200 due south; 60 μl/min)
Statistical analyses
Statistical analyses of Dna microarray spots were performed using ImageJ 1.51 southward. Illustrated data values are expressed as means ± standard deviation if non stated otherwise. Ratio values of fluorescent intensities between the original and copied microarrays were calculated past making use of the propagation of uncertainty. The supplementary movies S4 and S5 were created past using iii dissimilar ImageJ macros (Supplementary Software S6–S8).
Data Availability
The tiff images and SCORE datasets of the electric current study are available from the corresponding author on reasonable asking. Master and diploma theses are available as PDFs from the corresponding author on request.
References
-
Heller, M. J. Dna microarray technology: devices, systems, and applications. Annu. Rev. Biomed. Eng. 4, 129–153 (2002).
-
Bumgarner, R. Overview of Deoxyribonucleic acid Microarrays: Types, Applications, and Their Futurity. In Current Protocols in Molecular Biology Chapter 22, Unit 22.1. (John Wiley & Sons, Inc., 2013).
-
DeRisi, J. et al. Employ of a cDNA microarray to analyse factor expression. Nat. Genet 14, 457–460 (1996).
-
Fodor, S. P. et al. Light-directed, spatially addressable parallel chemic synthesis. Science (80-.). 251, 767–773 (1991).
-
Tindall, K. R. & Kunkel, T. A. Fidelity of DNA synthesis past the Thermus aquaticus DNA polymerase. Biochemistry 27, 6008–6013 (1988).
-
Lin, H., Sun, L & Crooks, R. Yard. Replication of a Dna Microarray, https://doi.org/10.1021/JA051914U (2005).
-
Lin, H., Kim, J., Sun, L. & Crooks, R. M. Replication of DNA microarrays from nothing code masters. J. Am. Chem. Soc. 128, 3268–3272 (2006).
-
Kim, J. & Crooks, R. 1000. Replication of DNA microarrays prepared by in situ oligonucleotide polymerization and mechanical transfer. Anal. Chem. 79, 7267–7274 (2007).
-
Akbulut, O. et al. Application of Supramolecular Nanostamping to the Replication of DNA Nanoarrays, https://doi.org/10.1021/NL0720758 (2007).
-
Yu, A. A. et al. High resolution printing of Deoxyribonucleic acid characteristic on poly (methyl methacrylate) substrates using supramolecular nano-stamping. J. Am. Chem. Soc. 127, 16774–16775 (2005).
-
Yu, A. A. & Stellacci, F. Stamping with high data density. J. Mater. Chem. sixteen, 2868–2870 (2006).
-
Yu, A. A. et al. Supramolecular nanostamping: Using Dna as movable type. Nano Lett. 5, 1061–1064 (2005).
-
Kim, J. & Crooks, R. M. Transfer of surface polymerase reaction products to a secondary platform with conservation of spatial registration. J. Am. Chem. Soc. 128, 12076–12077 (2006).
-
Hoffmann, J., Hin, S., Stetten, F., von Zengerle, R. & Roth, Yard. Universal protocol for grafting PCR primers onto diverse lab-on-a-chip substrates for solid-phase PCR. RSC Adv. ii, 3885 (2012).
-
Hoffmann, J., Trotter, Thousand., Stetten, F., von, Zengerle, R. & Roth, G. Solid-phase PCR in a picowell array for immobilizing and arraying 100 000 PCR products to a microscope slide. Lab Chip 12, 3049 (2012).
-
Gustafsson, M. Thousand. L. Surpassing the lateral resolution limit past a factor of two using structured illumination microscopy. J. Microsc. 198, 82–87 (2000).
-
Tasset, D. M., Kubik, M. F. & Steiner, W. Oligonucleotide inhibitors of human being thrombin that bind distinct epitopes. J. Mol. Biol. 272, 688–698 (1997).
-
Burger, J. et al. Low-Volume Label-Free Detection of Molecule-Protein Interactions on Microarrays by Imaging Reflectometric Interferometry. SLAS Technol. Transl. Life Sci. Innov. 22, 437–446 (2017).
-
Hölz, M. et al. High-Efficiency Contrary (5′→3′) Synthesis of Circuitous DNA Microarrays. Sci. Rep. viii, 15099 (2018).
-
Pirrung, M C., Laixin, W. & Montague-Smith, M. P. iii′-Nitrophenylpropyloxycarbonyl (NPPOC) Protecting Groups for Loftier-Fidelity Automatic 5′ → 3′ Photochemical Dna Synthesis, https://doi.org/x.1021/OL0069150 (2001).
-
Henderson, C. A., Rail, C. A., Butt, 50. E., Vincent, H. A. & Callaghan, A. J. Generation of small molecule-binding RNA arrays and their awarding to fluorogen-binding RNA aptamers. Methods, https://doi.org/10.1016/J.YMETH.2019.04.021 (2019).
-
Phillips, J. O. et al. High-density functional-RNA arrays as a versatile platform for studying RNA-based interactions. Nucleic Acids Res. 46, e86–e86 (2018).
-
He, M. et al. Press protein arrays from Dna arrays. Nat. Methods 5, 175–177 (2008).
-
Díez, P. et al. NAPPA as a Real New Method for Protein Microarray Generation. Microarrays (Basel, Switzerland) iv, 214–27 (2015).
-
Kilb, N. et al. Protein Microarray Copying: Easy on‐Need Poly peptide Microarray Generation Compatible with Fluorescence and Label‐Free Real‐Fourth dimension Analysis. Chem Bio Chem cbic.201800699, https://doi.org/ten.1002/cbic.201800699 (2019).
-
Norouzi, M., Pickford, A. R., Butt, Fifty. E., Vincent, H. A. & Callaghan, A. J. Application of mRNA Arrays for the Production of mCherry Reporter-Protein Arrays for Quantitative Factor Expression Assay. ACS Synth. Biol. 8, 207–215 (2019).
-
Krämer, Due south. D., Wöhrle, J., Rath, C. & Roth, G. Anabel: An Online Tool for the Real-Time Kinetic Analysis of Bounden Events. Bioinform. Biol. Insights thirteen, 117793221882138 (2019).
Acknowledgements
We would like to give thanks Holger Frey, Academy of Freiburg, IMTEK, Germany, Grouping of Prof. Rühe, for spotting of the original DNA microarrays. We give thanks the German Ministry for Art and Sciences (BMBF) for financial support and funding of the projects BioKopierer (FKZ 03VP01200) and AptaSELECT (01DL17007B).
Author data
Affiliations
Contributions
G.R. developed the initial idea and jointly refined it with S.D.G. and J.W. S.D.K., J.W. conceived and designed the experiments. S.D.M., J.W. analysed the data. P.A.Thou. designed and produced the wafer for primary cavity chip production. South.D.Chiliad. wrote the kickoff draft of the manuscript. South.D.K., J.W., P.A.Thou., G.U., Yard.R. contributed to the writing of the manuscript. G.U., G.R. hold with manuscript results and conclusions. G.R., One thousand.U., J.W., P.A.M. made critical revisions and approved the final version. All authors reviewed and approved the final manuscript.
Corresponding writer
Ethics declarations
Competing Interests
SDK, JW and GR are inventors of the presented method which is filed every bit pending patent (Percent/EP2019/062502).
Additional information
Publisher's annotation Springer Nature remains neutral with regard to jurisdictional claims in published maps and institutional affiliations.
Supplementary information
Rights and permissions
Open Access This commodity is licensed under a Creative Commons Attribution four.0 International License, which permits use, sharing, adaptation, distribution and reproduction in any medium or format, as long as you give appropriate credit to the original author(s) and the source, provide a link to the Creative Commons license, and indicate if changes were fabricated. The images or other 3rd party material in this article are included in the article'southward Creative Commons license, unless indicated otherwise in a credit line to the material. If material is not included in the article'south Creative Commons license and your intended use is not permitted by statutory regulation or exceeds the permitted apply, you will demand to obtain permission directly from the copyright holder. To view a re-create of this license, visit http://creativecommons.org/licenses/by/4.0/.
Reprints and Permissions
Nigh this article
Cite this commodity
Krämer, S.D., Wöhrle, J., Meyer, P.A. et al. How to copy and paste DNA microarrays. Sci Rep 9, 13940 (2019). https://doi.org/ten.1038/s41598-019-50371-one
-
Received:
-
Accepted:
-
Published:
-
DOI : https://doi.org/10.1038/s41598-019-50371-ane
Further reading
Comments
By submitting a comment you concur to abide by our Terms and Customs Guidelines. If you detect something calumniating or that does not comply with our terms or guidelines please flag it every bit inappropriate.
Source: https://www.nature.com/articles/s41598-019-50371-1
0 Response to "How to Copy Dna Over and Over Again"
ارسال یک نظر